This article is part of our Biotech Review of the Year - Issue 11 publication
CRISPR technology has come on leaps and bounds in the last decade.
After its discovery in 2012 and first application as a gene editing tool in mammalian cells the following year, improvements in genetic editing and delivery methods have allowed scientists to adapt CRISPR systems to potentially treat an array of diseases. Following the global-first approval of a CRISPR therapy by the MHRA in CASGEVY®, we take a look at a selection of the promising CRISPR treatments in the clinic.
What is CRISPR technology?
First, let’s revisit some basic principles. CRISPR stands for Clustered Regularly Interspaced Short Palindromic Repeat. This is the name given to the repeated DNA sequences that are found in bacteria. These sequences were originally derived from viral infections; the bacteria incorporates fragments of viral genome into their own genome, so that they could detect and destroy similar viral genes during future infections (thereby preventing viral proliferation).
This bacterial system has been manipulated to enable scientists to identify and edit specific genes. At a high level, CRISPR systems involve two components: (1) an enzyme which acts as molecular scissors to cut the genetic material (for example, Cas9) and (2) RNA which guides the enzyme to the target (for example, gRNA). These two components form a ribonucleoprotein complex. The RNA has two roles: a first spacer portion binds to the target gene and a second scaffold portion activates Cas9. Cas9 will bind the DNA next to the target gene provided it is adjacent to a specific sequence called a protospacer adjacent motif. This stabilises Cas9 which then makes a double-stranded cut in the DNA.
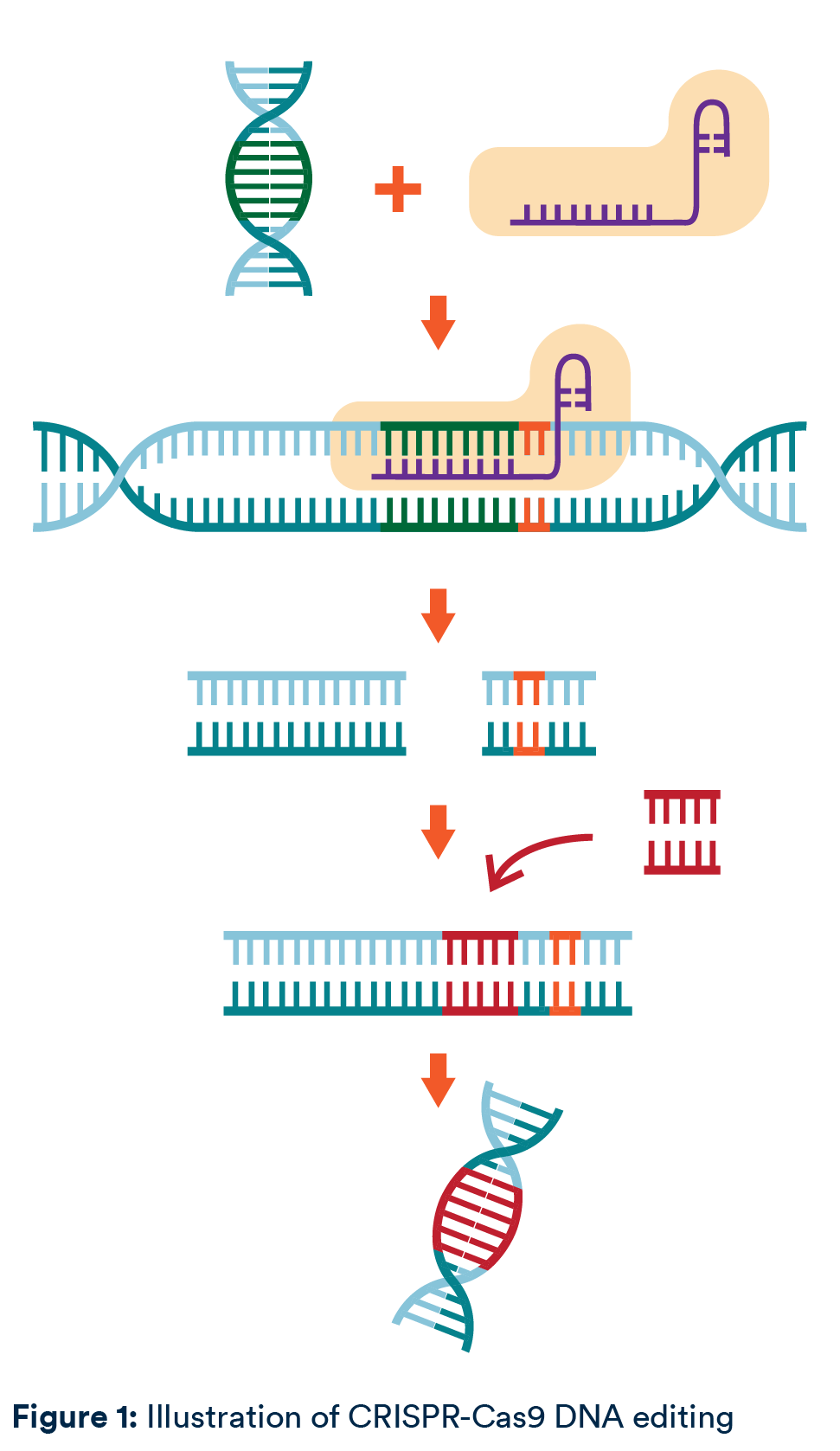
The double-stranded cut can be repaired randomly or with a template which encodes a new gene. The repair method has practical implications: DNA repaired randomly has the potential to give rise to unknown mutations, whereas use of templates has faced challenges with successful expression.
CRISPR/Cas9 was the first CRISPR gene editing method developed for practical applications and has generated a huge amount of interest. Indeed, the first CRISPR therapy to be approved involves CRISPR/Cas9, as do many of the treatments in clinical trials. However, since 2012, different enzymes to cut genetic material have been characterised, other types of RNA can be used and new genome editing techniques have been developed to limit off-target activity and unintentional mutations. CRISPR/Cas9 is now just one tool in the CRISPR-tool box.
CASGEVY® approval
On 16 November 2023, Vertex Pharmaceuticals, in collaboration with CRISPR Therapeutics, obtained the first regulatory approval in the world for a CRISPR-based therapy from the MHRA1. CASGEVY®, which contains exagamglogene autotemcel (exa-cel) as its active substance, is an autologous, ex vivo CRISPR/Cas9 gene-edited cell therapy authorised for the treatment of sickle cell disease and beta thalassemia.
Both sickle cell disease and beta thalassemia are caused by (slightly different) mutations in the beta-globin gene which results in dysfunctional adult haemoglobin (HbA). HbA is essential for carrying oxygen in red blood cells. CASGEVY® treats these disorders by increasing amounts of foetal haemoglobin (HbF). Typically, during gestation, foetuses produce HbF. Shortly after birth, production of HbF is repressed and a switch to HbA occurs. CASGEVY® seeks to restore expression of HbF in patients that are unable to produce normal levels of functional HbA. The patient’s own stem cells are harvested and CRISPR/Cas9 is used to silence the gene that represses the production of HbF. The patient then undergoes a stem cell transplant, whereby the edited stem cells are reintroduced. Like many CRISPR therapies, it is hoped that this will be a one-time treatment.
The approval of CASGEVY® by the MHRA represents a world first. CASGEVY(R) has subsequently been approved for sickle cell disease by the US FDA on 8 December 2023, and it is possible that approval will follow for beta thalassemia by 31 March 2024. Approval in the EU for both sickle cell disease and beta thalassemia is expected imminently following a positive opinion of the EMA’s CHMP on 14 December 2023.
Despite this landmark achievement, Vertex will now need to navigate pricing discussions with local authorities. Following approval, there has been speculation in the press that this one-off therapy could cost around the £1 million mark or more. In the UK, whether the NHS is willing to foot that bill remains to be seen. That said, it seems as though NICE has anticipated (perhaps unsurprisingly) the interest in this new type of therapy, having already initiated assessments to appraise the clinical and cost effectiveness of exa-cel in both indications2.
Platform technologies in the pipeline
In the clinical pipeline, Intellia’s CRISPR/Cas9 therapy NTLA-2001 is being developed in collaboration with Regeneron to treat transthyretin amyloidosis (ATTR)3. Transthyretin is a protein produced in the liver, which is encoded for by the TTR gene. Mutations in the gene cause transthyretin to misfold, which can cause neuropathy and/or cardiomyopathy. Unlike CASGEVY®, NTLA-2001 is an in vivo treatment, whereby patients are delivered an intravenous infusion of Cas9 mRNA and gRNA encapsulated in a lipid nanoparticle. These lipid nanoparticles accumulate in the liver cells, whereby the Cas9 mRNA is translated into the functional Cas9 enzyme and forms the ribonucleoprotein with the gRNA. CRISPR/Cas9 then knocks out the TTR gene, thereby reducing levels of misfolded transthyretin in circulation. A phase 3 trial was due to start by the end of 20234.
Excitingly, Intellia’s technology may represent the first so-called “platform” CRISPR therapy, insofar as small changes to the gRNA result in the ability to treat a different disease. By changing only the nucleotide bases in the gRNA, Intellia’s NTLA-2002 therapy is able to target and knock out the gene associated with causing hereditary angioedema. Hereditary angioedema is an inflammatory disease which presents as recurring, unpredictable swelling which can require medical intervention to prevent asphyxiation. Like the therapy for ATTR, NTLA-2002 uses lipid nanoparticles to deliver CRISPR/Cas9 to the liver, where it targets the faulty gene. A phase 2 trial is currently recruiting5.
CRISPR in cell therapy
The advent of personalised medicine has provided tailor-made treatments for individuals based on their specific disease and risk profile. Many personalised therapies involve editing the patient’s own cells (so-called cell therapy). Chimeric antigen receptor-T cell (CAR-T) immunotherapy is an example of this that utilises the patient’s own immune system to target blood cancers. T cells are collected from the patient (in other words, autologous cells) and engineered to express a chimeric antigen receptor that is capable of recognising tumour-antigen. These T cells are expanded and, following chemotherapy to reduce the patient’s remaining immune cells, administered to the patient. However, there are inherent challenges to such an individualized treatment. The manufacturing process takes time, is expensive and successful manufacture is not guaranteed. Sometimes the patient’s immune cells are not potent enough, the recombinant engineering simply fails, or it takes so long that the patient can no longer receive treatment. Further, even if CAR-T administration is successful, negative regulatory systems (that are part of the patient’s immune system) can put the brakes on the T cell response.
CRISPR is now being investigated to make improvements to autologous CAR-T, for example to make the cells more resistant to T cell exhaustion by removing the cell-surface protein PD-1. Tumours can trigger T cell exhaustion, which essentially reduces the
anti-tumour T cell response, by binding to PD-1 on the T cell.
To tackle some of the logistical issues facing autologous CAR-T, CRISPR is also being exploited to generate “off the shelf” universal CAR-T therapies. T cells from donors (in other words, allogeneic cells) are edited with CRISPR to attempt to make them resistant to rejection by the patient’s immune response, reduce T cell exhaustion by knocking out PD-1 and, of course, to target the tumour antigen. Allogeneic CAR-T offers promise in that it does not depend on the quality of the patient’s own immune cells and should, in theory, be available more quickly to treat patients (hence, “off the shelf”). Both of these factors are particularly important in patients with late-stage cancer.
CRISPR in infectious disease
So far, we have looked at ex vivo and in vivo therapies for monogenic disorders and CRISPR cell therapies in oncology. Demonstrating the versatility of this technology, CRISPR is being explored by Locus Biosciences to genetically engineer bacteriophage – viruses that infect bacteria – to treat multi-drug resistant E. coli urinary tract infections6. The bacteriophage are enhanced to deliver CRISPR/Cas3 to the bacteria. Whereas Cas9 causes a double-stranded break, Cas3 degrades the genetic material, destroying the bacterial genome. In addition to acting as a viral-delivery vector for CRISPR/Cas3, the bacteriophage itself possesses lytic activity, meaning that treatment attacks the bacterial infection on two fronts. A pervasive issue with current antibiotics is that many of them are broad spectrum; the infectious bacteria may be destroyed, but so will the patient’s “good” bacteria. CRISPR enhanced phage can be tailored so as (1) to infect only the harmful bacteria; and (2) the CRISPR/Cas3 construct only destroys the harmful bacterial genome. Locus Biosciences’ LBP-EC01 product, which comprises a cocktail of CRISPR-enhanced phage, is currently in Phase 2/3 trials7.
New editing technologies
The prospects of CRISPR/Cas9 therapies in the clinic are hugely promising. That said, there are of course inherent risks with editing genetic material. Causing double-stranded breaks and introducing unintentional mutations, or causing off-target double-stranded breaks could lead to potentially harmful side effects. Fortunately, even more precise methods of editing are on the horizon. An example of this is base editing. It is now possible to introduce single genetic edits without causing a double-stranded cut. This is done by an inactive Cas9 or Cas9 nickase fused to an enzyme that can directly change the intended nucleotide base.
Through this method, off-target mutations are less likely. In November 2023, Verve Therapeutics announced interim data from its Phase 1b trial for its VERVE-101 product to treat heterozygous familial hypercholesterolemia, a condition which causes high cholesterol. VERVE-101 comprises a base editor and gRNA packaged in a lipid nanoparticle. These are taken up by liver cells, where the base editor switches one nucleotide base in the PCSK9 gene to deactivate it. According to the interim data, VERVE-101 led to reductions in LDL cholesterol.8 The clinical trial is ongoing.
Looking ahead
It is hard to overstate just how far CRISPR has come in the past decade. From inception as a brand new technology in 2012, to authorisation as a medicinal product in CASGEVY® in 2023, the rate of advancement has been astounding. This technology has the potential to transform medicine, across not only monogenic and complex genetic disorders, but also in infectious diseases and oncology. Of course, it is not all smooth sailing from here. Approval of the first CRISPR therapy does not equate to access. Potentially challenging discussions on pricing and health equity lie ahead. It also cannot be assumed that every CRISPR therapy currently in the clinic will yield an authorised treatment. Looking further ahead, more data is required to assess any undesirable long-term effects of in vivo genetic editing.
All that being said, it is undeniable that the future is promising. CRISPR technologies represent a seismic shift in precision medicine, and with advances in genetic engineering the potential is almost limitless.
=======================
1 https://www.gov.uk/government/news/mhra-authorises-world-first-gene-therapythat-aims-to-cure-sickle-cell-disease-and-transfusion-dependent-thalassemia
2 https://www.nice.org.uk/guidance/indevelopment/gid-ta11249
https://www.nice.org.uk/guidance/indevelopment/gid-ta11250
3 https://www.intelliatx.com/pipeline/
4 https://clinicaltrials.gov/study/NCT06128629?term=ntla-2001&rank=2
5 https://clinicaltrials.gov/study/NCT05120830?term=ntla-2002&rank=1
6 https://www.locus-bio.com/locus-biosciences-announces-first-patient-treated-inthe-eliminate-registrational-phase-2-3-trial-of-lbp-ec01-for-urinary-tractinfections/
7 https://clinicaltrials.gov/study/NCT05488340?term=LBP-EC01&rank=2
8 https://ir.vervetx.com/news-releases/news-release-details/verve-therapeuticsannounces-interim-data-verve-101